Why sauna designers should care about the Law of Löyly
Law of Löyly initially appeared as a witty remark in the Finnish literature over 60 years ago. As the interest in saunas, their design and optimization has surged, we’ve seen people both in Finland and abroad question and go against this guideline. Should everyone just forget this old joke, or are there valid reasons to continue observing the law? Saunologia guest writer Walker Angell of Trumpkin’s Notes discusses the physics that enforce the law and the attempts to devise ways around it.
In 1960 Sakari Pälsi gave us what are perhaps the most immortal words in the world of sauna design. Often paraphrased as “Feet Above The Stones” and called variously ‘The Law of Steam’, ‘The First Law of Löyly’ or ‘Pälsi’s Law’ (in Finnish ‘Löylyn laki’ or ‘Ensimmäinen löylyn laki).
“The point is that when you sit on the bench, make sure the steam stones are at the level of your toes and the ceiling is just within reach of a birch whisk.”
– Sakari Pälsi, ‘Sauna’, 1960
This was the result of his exploration of saunas over the prior decades and noticing that saunas with good steam and good löyly always followed ‘the law of steam’, and those that did not follow the law had ’poor löyly’.
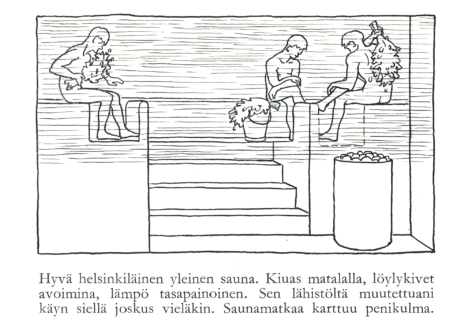
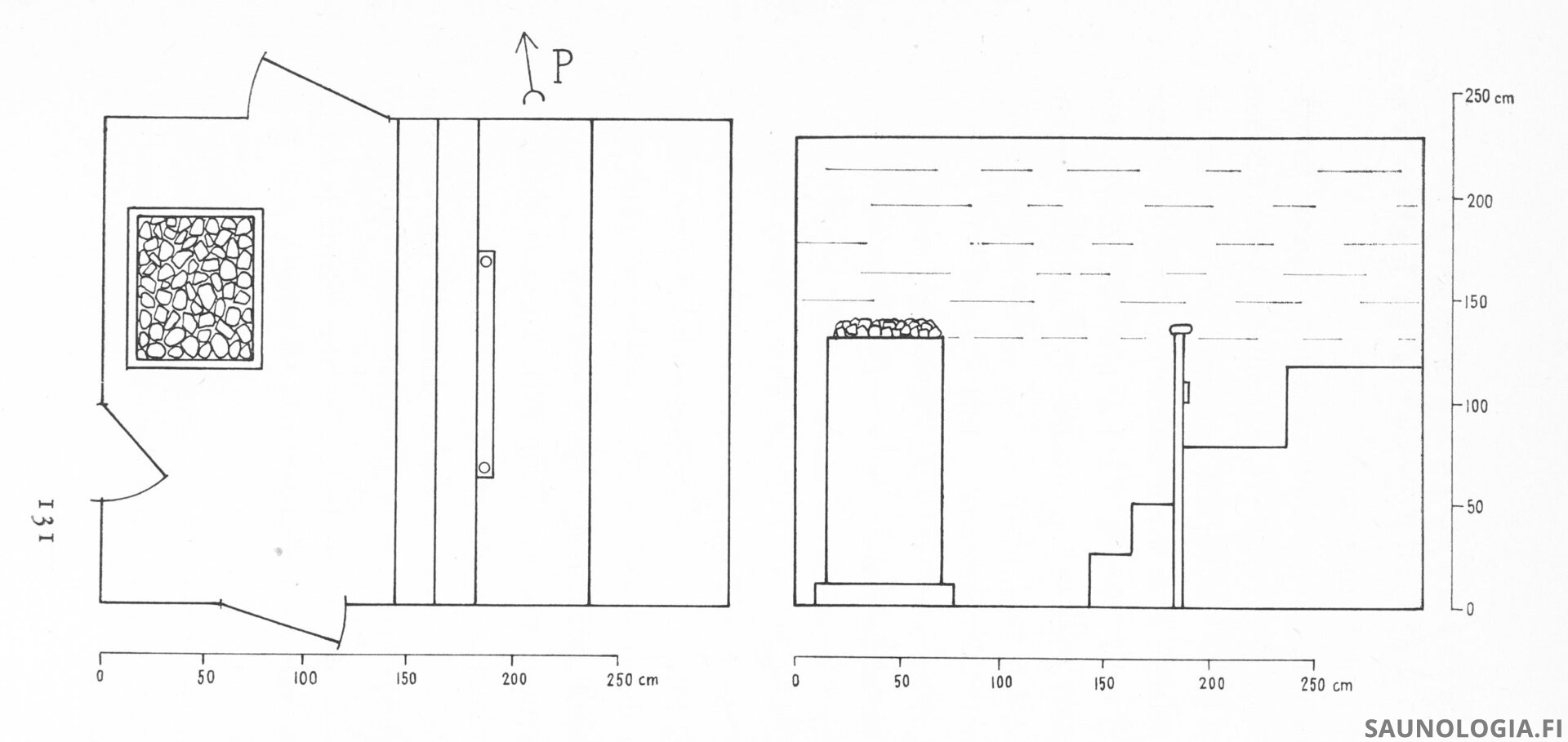
This wasn’t a new idea though. Pälsi noted that sauna builders had clearly known this for a while and that with good builders “the law of steam is followed almost unnecessarily precisely”.
Professor Pälsi was a known archeologist and ethnographer, who worked at the Finnish National History Museum most of his career. He is not considered a sauna specialist in Finland. Finnish sauna was one of the topics he wrote a book about in the final years of his life, published at the age of 78, five years before his death.
Still For Today?
A question for us today is if this is still valid? Do we need to follow the law of steam to have a good sauna or does newer technology allow us to circumvent Pälsi’s 65-year-old law?
In particular, open-sided mesh tower heaters have become popular, in Finland and elsewhere. The exposed stones and overall size and shape are aesthetically appealing. Designed properly they emit very little radiant heat, produce a good convective loop and good steam. There’s a lot to like about them.
But can your feet be below the top of the stones of these tall heaters as those selling them tell us?
Evidence in favor
Lassi and I along with others have considered this in numerous saunas when we’ve visited them and whenever possible have taken hard measurements. What we’ve learned is that good temperatures (chart below) can indeed sometimes extend to a bit below the top of the stones with open sided mesh towers, as demonstrated by Lassi’s test of 3 Narvi heaters.
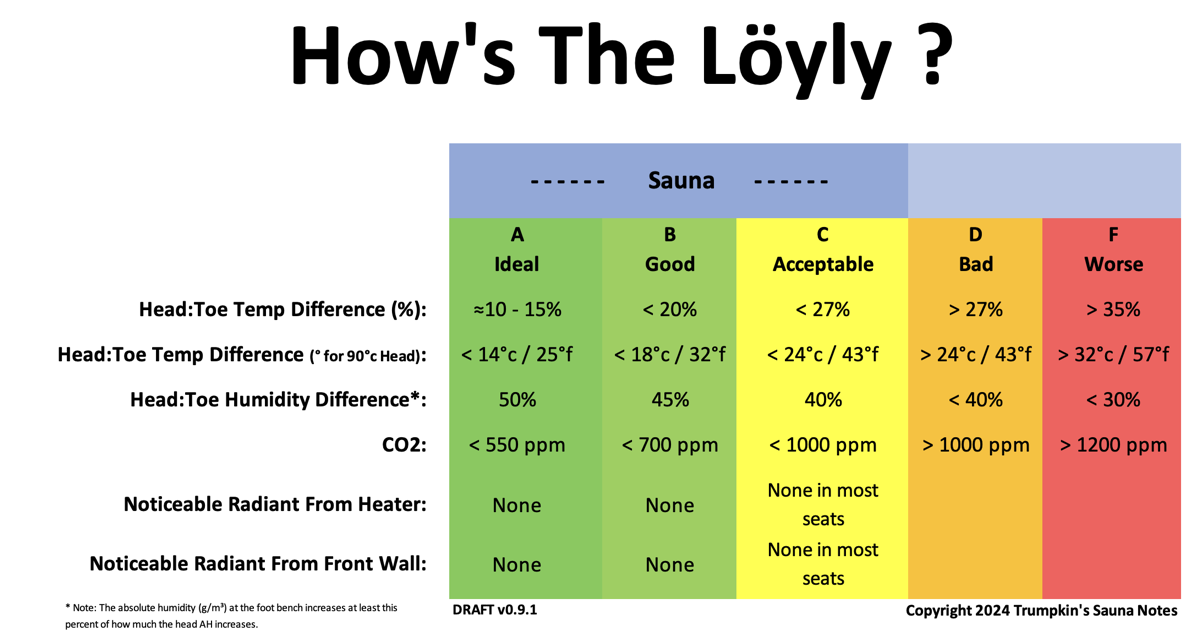
Steam however seems to never descend below the top of the stones, except in a few instances of larger spaces like Pereensaari where the heater and benches are very high within the space or in some larger smoke saunas where there may be a cardioid affect that allows it to go lower when very far from the heater. (Note: Pereensaari also uses a pair of Saunasampo heaters with internal blowers that may help).
For me one of the best examples of the stubbornness of steam is at Lakeuden where Lassi noted that good temps did extend below the top of the stones of the large Sydän heater, but steam surprisingly did not.
Some of us have also tried various experiments, like Ted’s Excellent Hollowcore Adventure, to get steam to descend lower. They’ve helped, but by only a limited amount.
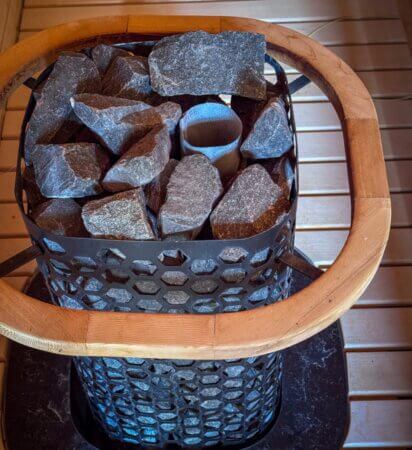
So, what gives? Let’s explore a few potential explanations for this phenomenon.
The Anatomy of Steam
When we throw water on the stones in our sauna, that water is quickly heated on the surface of the stones until it reaches its boiling point when it is then vaporized and becomes what we commonly call steam.
Steam is water in its gaseous phase – water vapor. The water that had been comprised of a bunch of H2O molecules all bound together to create a liquid is, thanks to some extra heat energy, now unbound or free molecules – a gas. The hydrogen bonds that had held the molecules together to form a liquid no longer do.
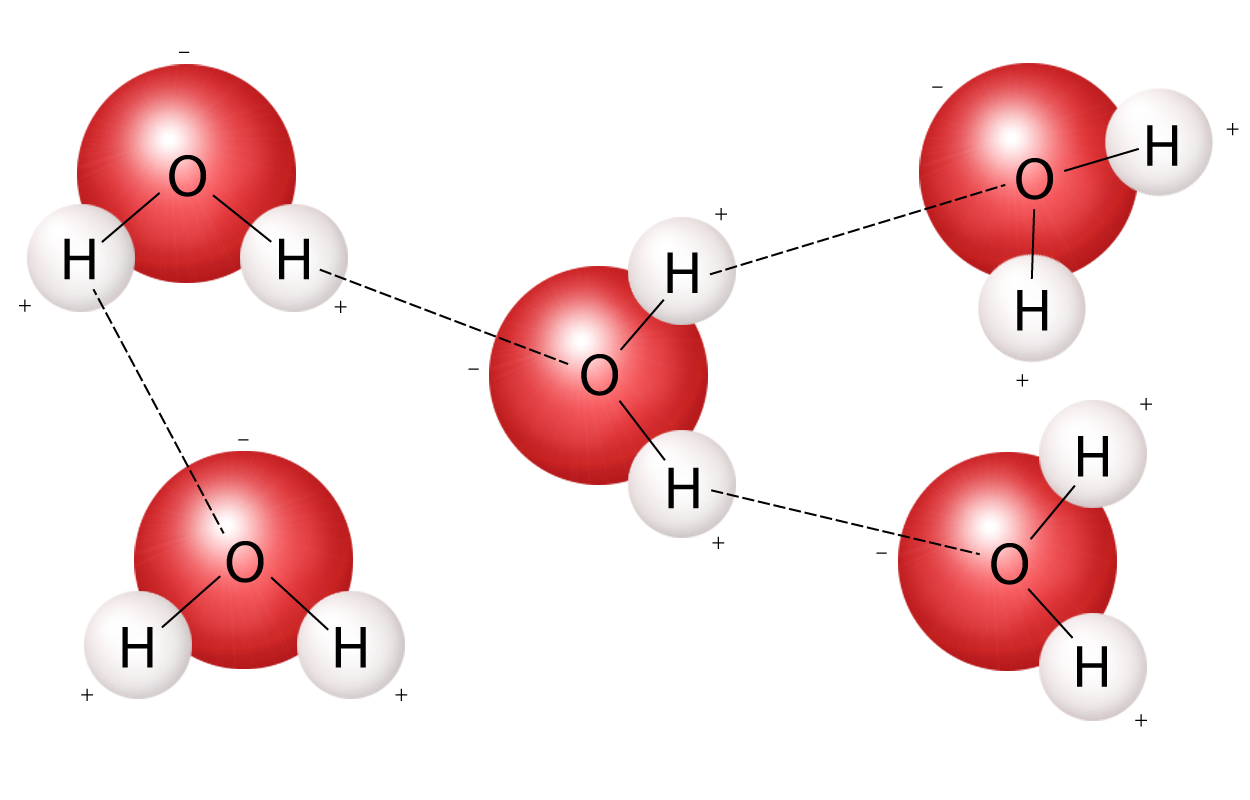
To get an idea of the size of one of these H2O molecules: A human hair has a diameter of about 60 µm (microns). A droplet of fog (liquid water), like in a steam room, averages about 7 µm (though as small as 1 and as large as 50 µm) so about 1/9 the diameter of a human hair.
The smallest thing that we can resolve with our eye is about 100 µm. We can see a hair only because of its length and fog only because there are gazillions of these little droplets close together. And note that fog is not a gas but droplets of liquid.
There are about 17 trillion H2O molecules in each one of those 7 µm fog droplets.
How about 1.5 sextillion H2O molecules in an average drop of water that might boil to death on a stone in our heater. That would be 1,500,000,000,000,000,000,000 molecules per drop. Times about 33,000 drops per 330ml ladle of water.
That is steam. Water deconstructed.
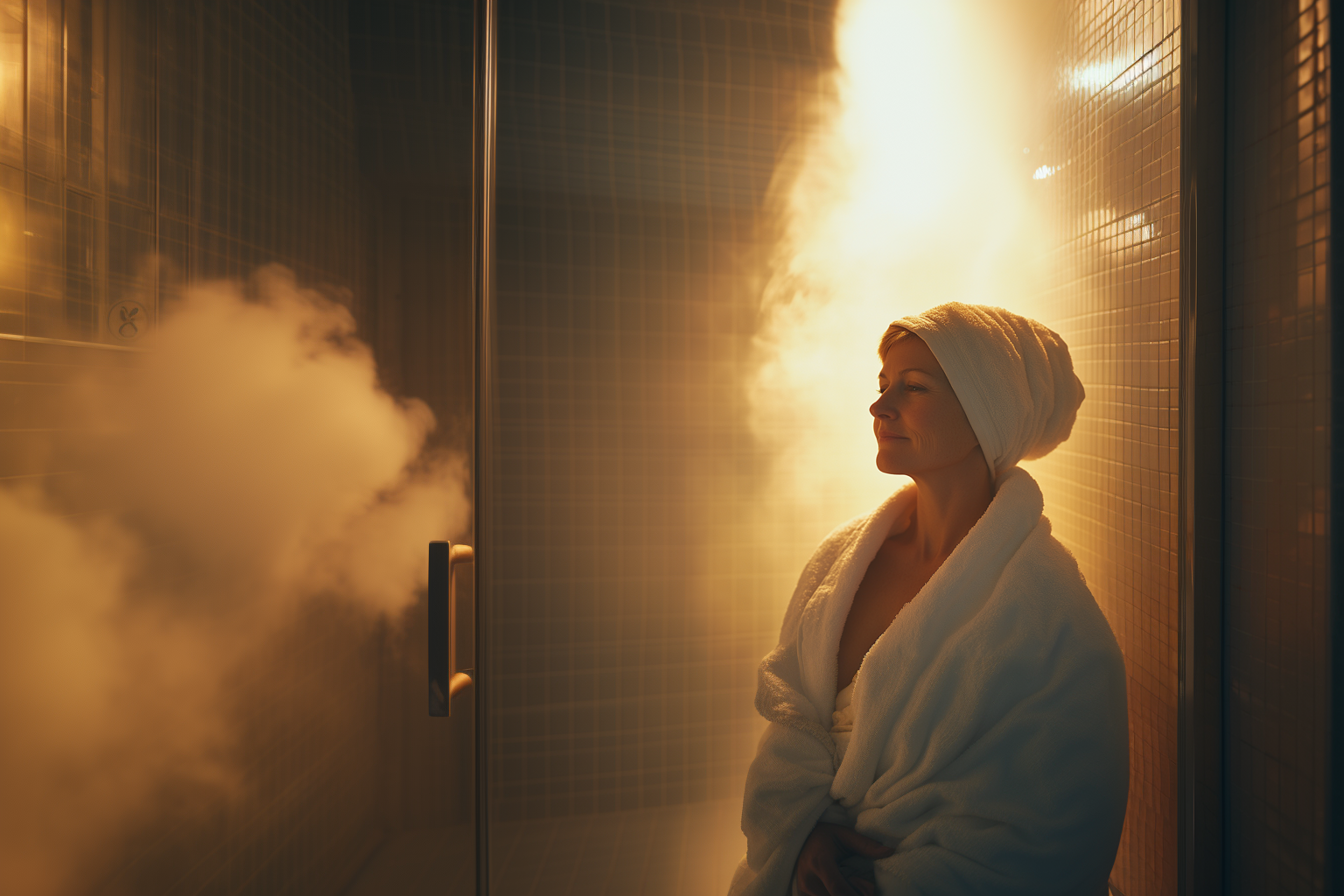
How steam behaves in sauna
So, these gazillions of individual H2O molecules we call steam begin to rise from the stones where they were freed. There’s a flow of air that rises up from the heater, across the ceiling away from the heater, down the far walls and then returns to just above the heater – a convective loop. Many of these molecules kind of follow most of this loop, while a few spread out directly towards us.
When these molecules collide with our cooler skin, or anything that’s colder than the dew point, they condense back into liquid water. The cooler air at the surface of our skin for instance, cools the molecules which results in their moving slower. They no longer have the energy to remain free from each other and so the H bonds that were broken when they became a gas are reformed. About 1,500,000,000,000,000,000 molecules, give or take a few trillion, come together to form each droplet of liquid condensation on our skin.
Here’s the fun part. The energy that was used to break the H bonds and free the molecules from each other didn’t just go poof. Remember, conservation of energy.
First, the energy used to heat the water we threw on the stones up to boiling, raised the temperature of the water, from perhaps 30°c to about 100°c. That energy was simply transferred from the stones to the water. This is what we call sensible heat.
Then, additional energy (heat) from the stones is used to convert the water to steam, to break the H bonds and free the molecules. This is called a phase change (liquid to gas) and does not increase the temperature of the steam molecules. But the energy is still there. It became latent heat, or hidden heat, amongst the H2O molecules. This is called the latent heat of vaporization or enthalpy of vaporization. It’s there as heat potential.
When those molecules condense back into water in our skin, they release that latent heat. It’s no longer part of the steam and must go somewhere, and somewhere is us. That’s why steam feels so hot.
One cool thing about the latent heat of steam is the total disconnect between the latent heat that we feel in our skin and air temperatures. Frequently there’s no change in air temperatures, sometimes there’s a small increase and sometimes a small decrease. Yet we feel a very significant increase.
Interestingly though, when we make steam, the air temperature over the heater …declines.
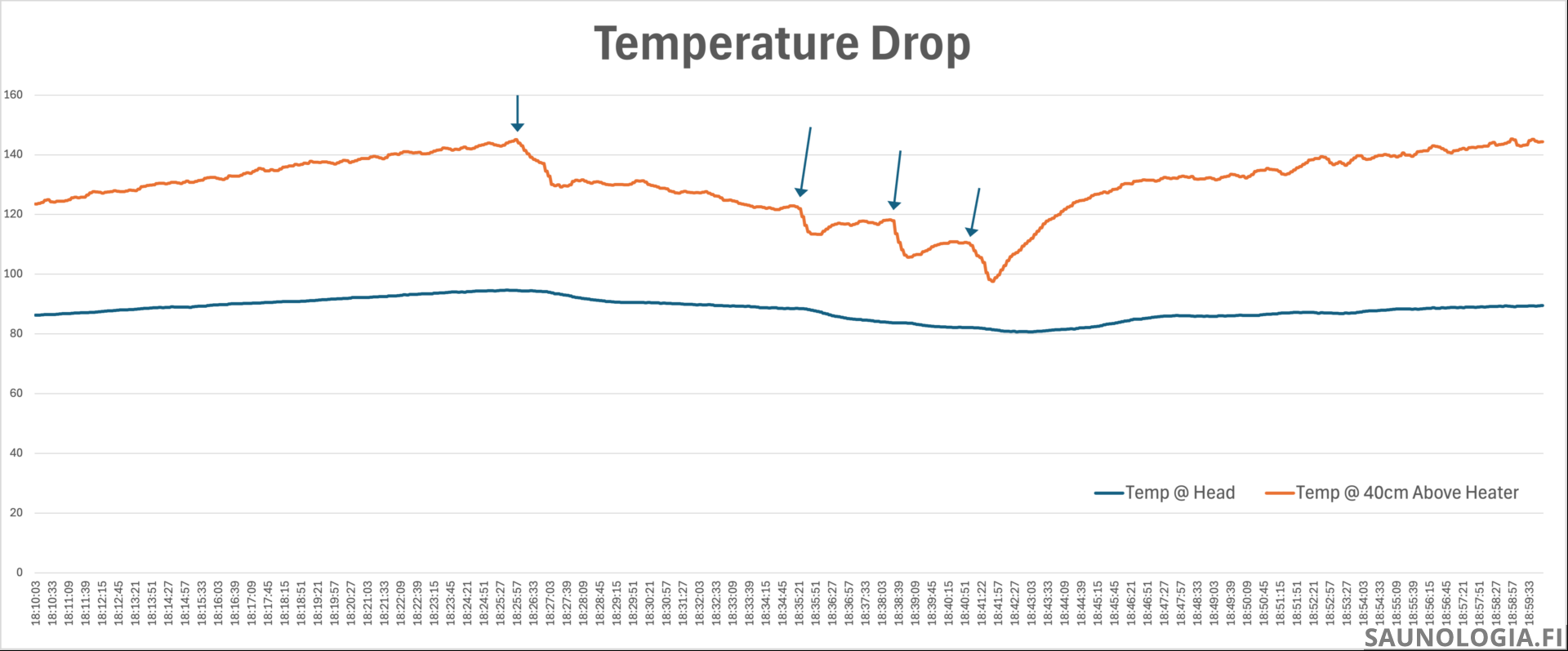
This is likely caused by a combination of things: 1) The energy transferred to the steam as latent heat of vaporization is energy that is no longer in the stones to heat the air, 2) Heat is transferred from the stones to the water to heat it up to 100°c is no longer available to heat the air, and 3) the cooler 100°c steam mixed with the hotter air results in a cooler gas mixture. And yeah, that steam that feels so hot when it hits our body is actually cooler than the air rising from the heater.
Note that the heater was in its OFF phase of the cycle the first steam and turned on just before the second.
Skin Penetration – One more quick bit about steam’s journey to us and why it can feel so much hotter than the air. Steam molecules (H2O) are smaller than most of the molecules that make up the air (78% nitrogen or N2 and 21% Oxygen or O2) that carries convective heat to us. So steam can penetrate our skin with heat easier, deeper and quicker. It condenses and releases its latent heat deeper in our skin which feels hotter than the same heat on the surface of our skin. That heat also gets to our muscles faster for localized effect.
Note: I am using the term ‘steam molecule’ for ease of reading. I’m referring to any free H2O molecule in a sauna, e.g., in its unbound gaseous state, of any temperature and with latent heat potential.
The ‘any temperature’ part may be problematic as some people define steam as only molecules with a temperature of 100°c or greater. In other words, once the molecule cools to anything below 100°c it is no longer called steam but simply water vapor. There is however, no actual change that takes place other than a decline in temperature. In this instance there is nothing magical about 100°c as there is with vaporization. It is still an unbound molecule and still retains the same latent heat potential that it gained from the endothermic vaporization process.
Why Does The Steam Stay Above The Stones?
Why does the steam seem to always stay above the stones? And why does it not seem to respond to downdraft ventilation as well as hot air does?
Spoiler – I don’t think we know for 100% certain. (But we’ve got some ideas.)
Buoyancy – First is buoyancy, here steams ability to float in air. Like a boat in water, steam molecules in air are more buoyant than air molecules – they displace a lot more air for their weight. So, some 90°c steam molecules and some 90°c air molecules at the same strata fight for space and the steam molecules get the upper bench and push the air molecules down. Steam 1 – Hot Air 0.
Energy / Heat Retention – Steam molecules have a higher heat capacity than air molecules, so steam molecules are slower to lose their heat. If 10 steam molecules and 10 hot air molecules are, thanks to buoyancy, hanging around the 90°c strata together enjoying some Finnish long drinks – the steam molecules will lose perhaps 0.1°c to 89.9°c and sink down a tiny bit but in that same amount of time the hot air molecules will lose maybe 0.2°c to 89.8°c and sink down twice as far. Steam 2 – Hot Air 0.
Density – When steam is first created and all jammed up against the ceiling there are a lot of molecules per cubic meter of space. If we stick our head or hand up here, a bunch of molecules will run into our skin and release their latent heat.
Yeah, it feels hot! How hot steam feels is based on the density of the molecules – how many are in a given amount of space. The more molecules there are to condense in our skin and release their latent heat, the hotter the steam feels. Releasing steam also causes perceptible movement of air, also enhancing the heating effect of air convection.
As the steam spreads downward and begins to occupy a larger space, the density decreases, or decays. If we’ve 10 gazillion molecules in the upper 20 cm and then it spreads down a bit so it’s filling the upper 40 cm, then we’ve about half the density. We had 5 gazillion molecules per cubic meter and now only 2.5 gazillion per cubic meter. Same number of molecules but spread out more.
Consequently, we’ll experience about half as much heat per square cm of skin. The further down it spreads the less dense it is and the less hot it feels.
We can see this in the following chart.
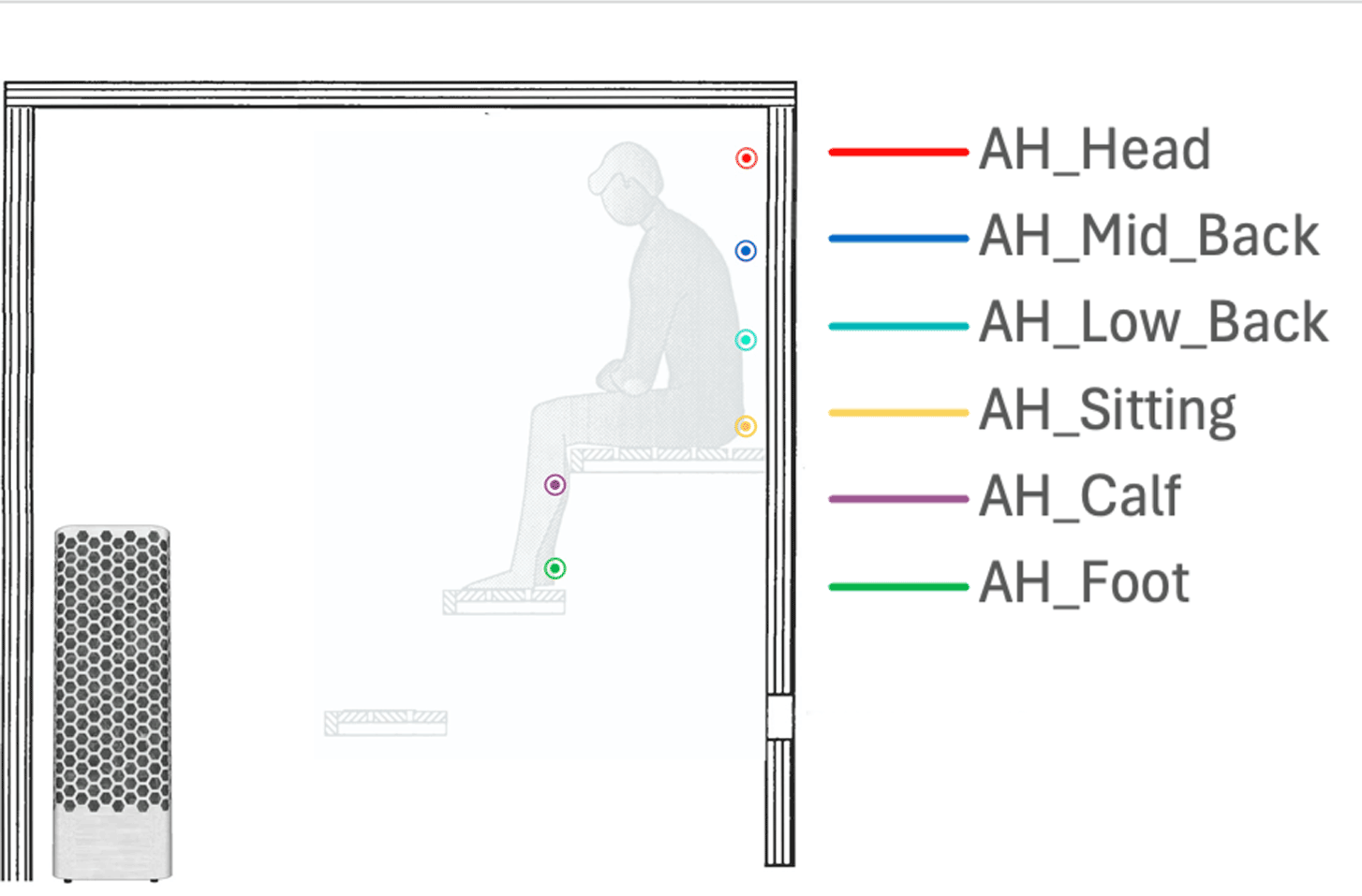
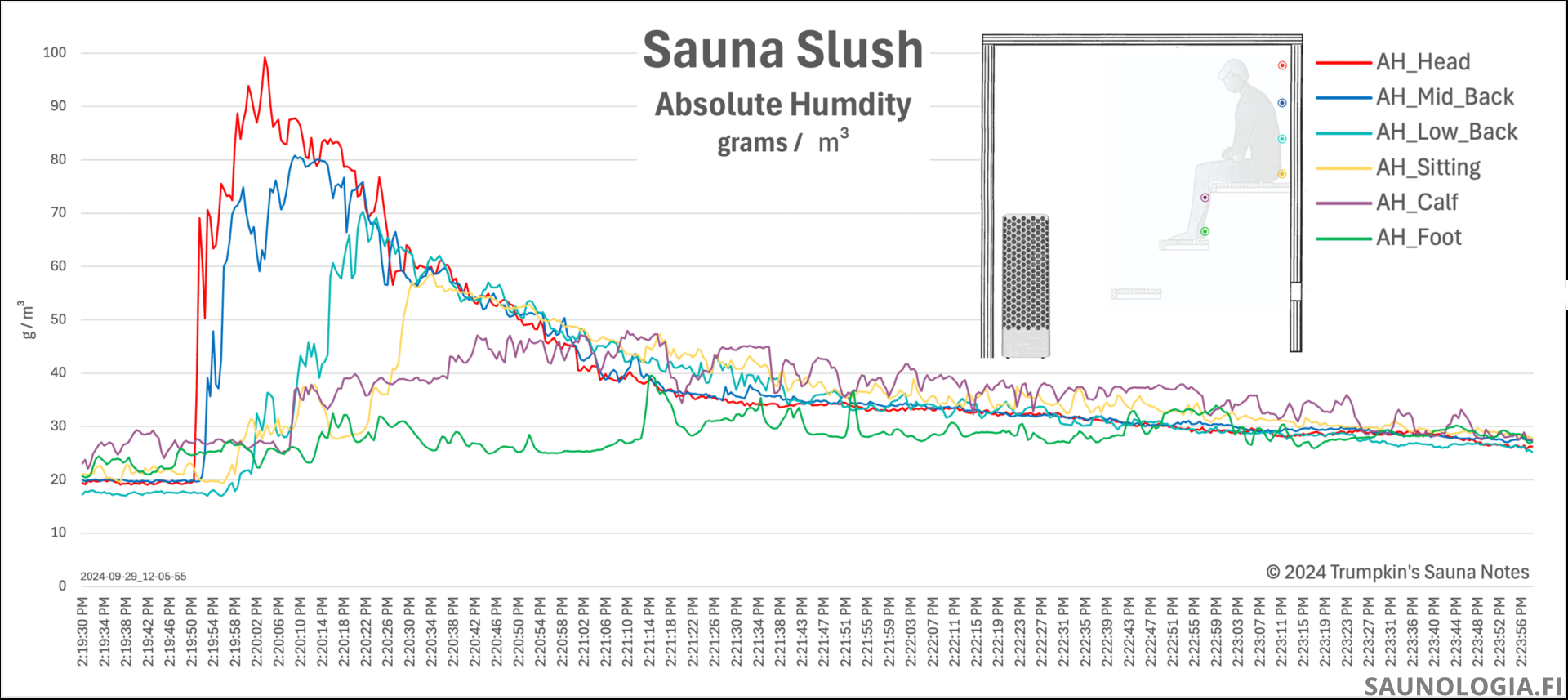
Time – Over time, more and more of our steam molecules encounter something that results in condensation. This is most often our skin but could be a cool piece of metal or even a bench.
They may also encounter air that’s below the dew point which can cause them to condense into a very fine fog. This fog can be invisible to our human eyes or like what we see when we open a door to outside and moist steamy air hits cold air and creates a fog that appears like smoke.
So, we start with 10 gazillion molecules and after 30 seconds 3 gazillion have condensed, so we’re left with only 7 gazillion steam molecules.
To sum up so far… Buoyancy and Heat Retention cause the molecules to remain higher up and sink very very slowly. As they do cool and sink, they occupy more space resulting in a decrease in Density while at the same time we’re losing molecules to Condensation resulting in even less Density.
Understanding the convective loop
Now let’s take a closer look at the convective loop, the role it plays with air and steam and importantly, why it seems to stay above the stones.
First, we have a lot of very hot air exiting the heater just above the top of the stones.
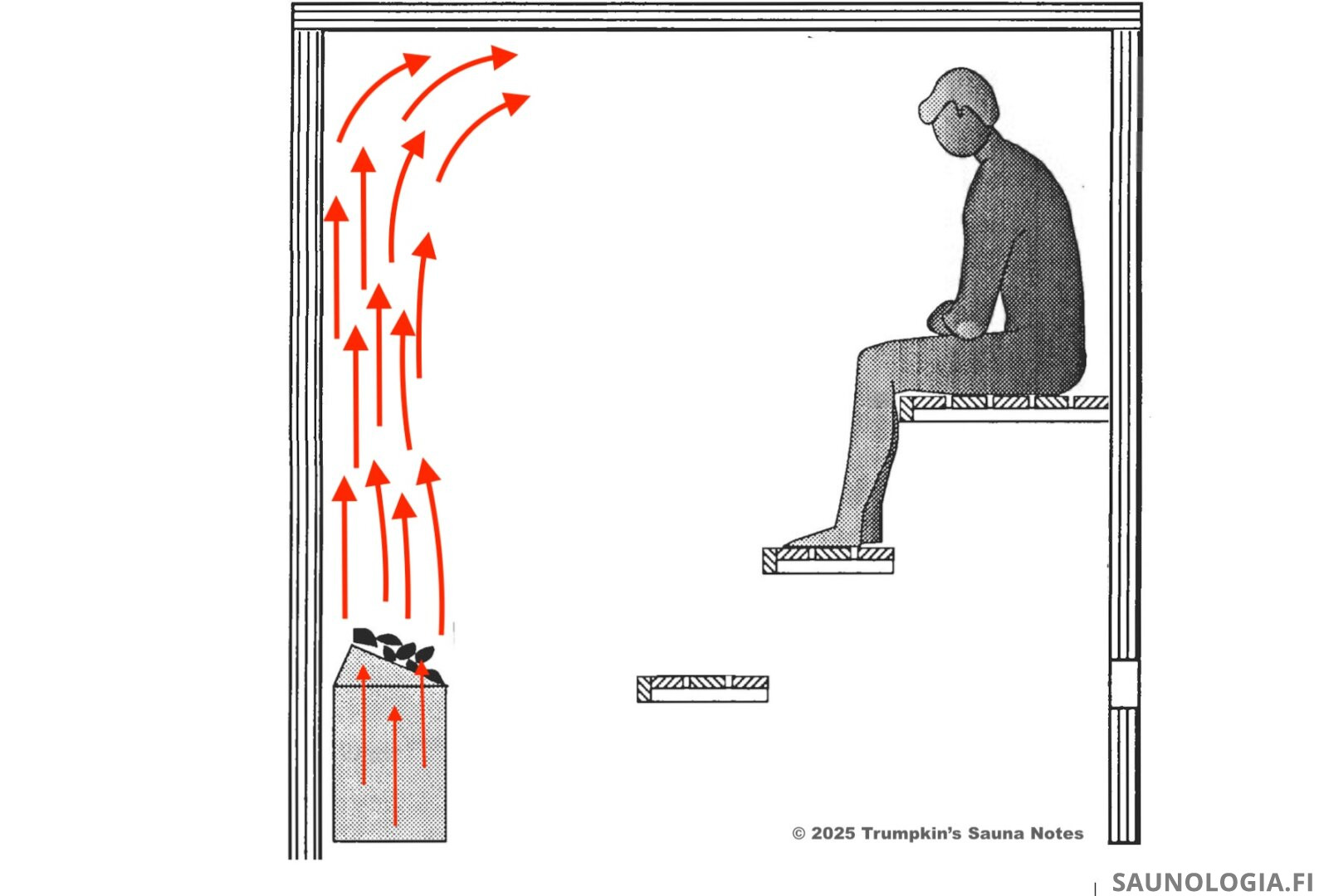
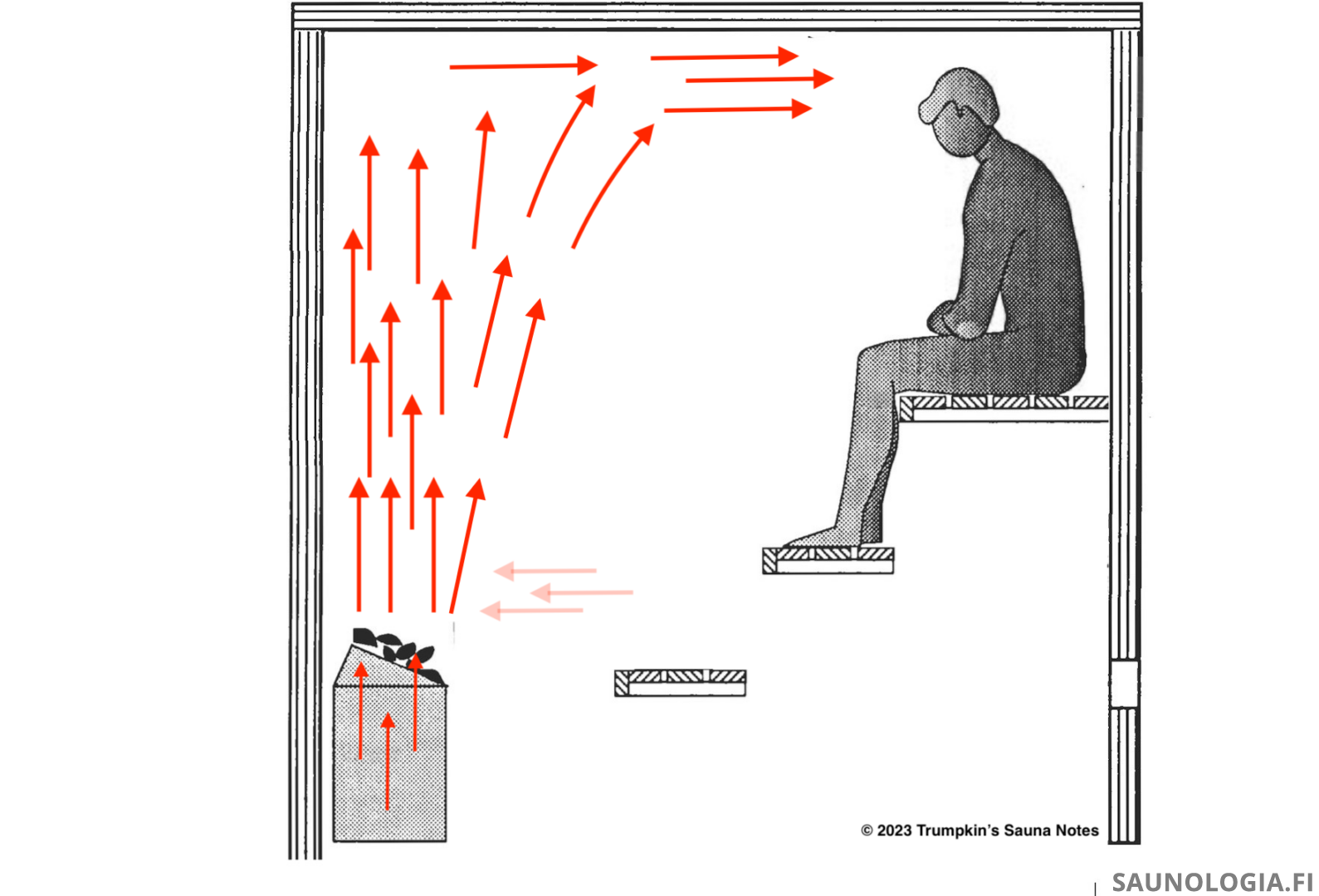
The air above the heater now wants to rise faster than the air inside the heater which creates a vacuum in this space just above the stones. We have perhaps (guesstimate) 1 liter/sec of airflow up through the heater but then 5 l/s above. This 4 l/s deficit creates a vacuum that is filled by pulling in air from the space next to it–from the same strata (light red arrows)–which in turn creates a vacuum there that is likewise filled with air from the same strata further to the right in our photo. It pulls from the same strata because it’s kind of lazy–pulling air from higher or lower involves too much energy. The same strata is the path of least resistance.
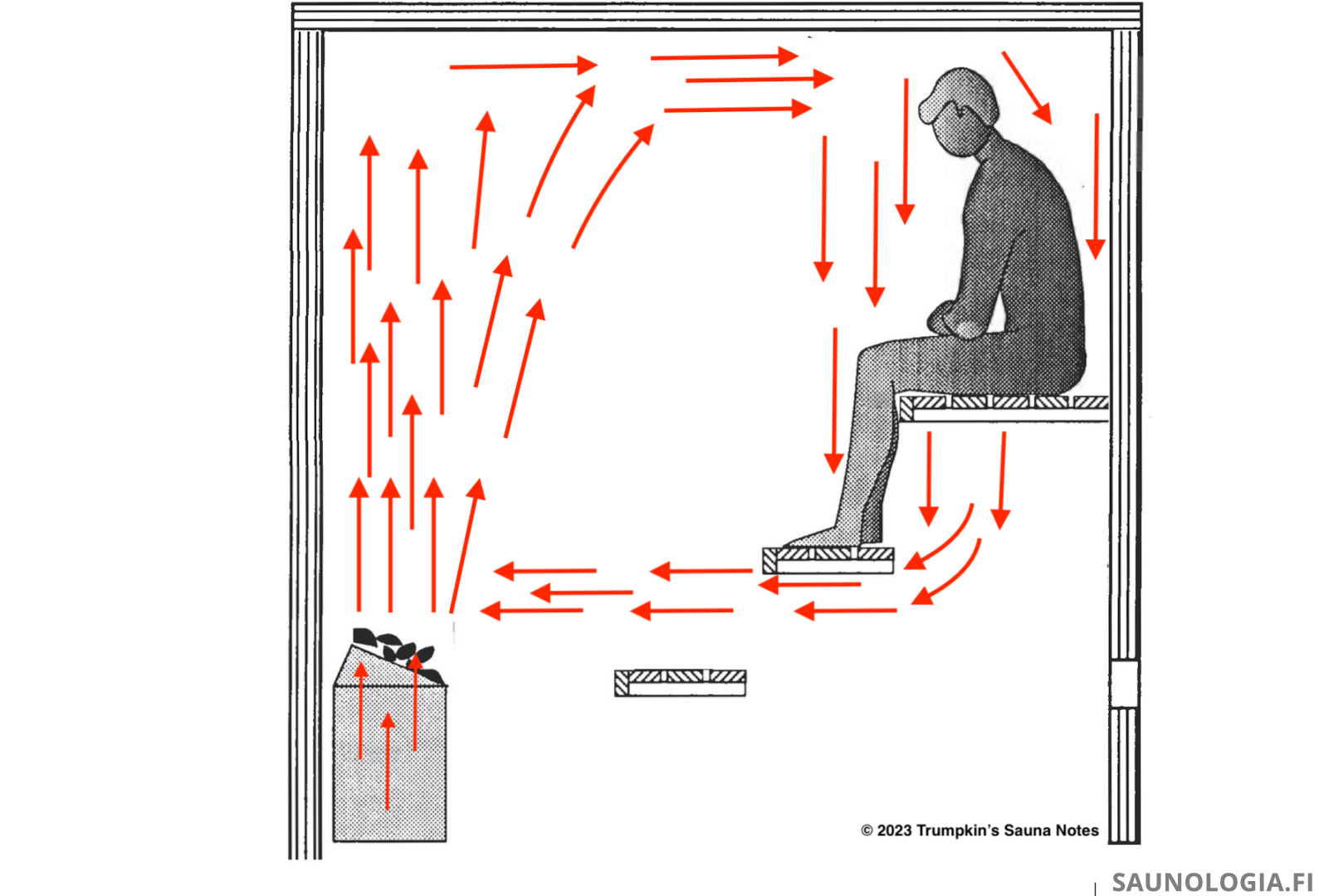
At the bottom of the convective loop then we’ve got the return air current flowing back towards the area just above the heater stones. Steam molecules that make it down this far are happy to ride along. Just like air, they’re staying at the same strata. So, they flow back to just above the heater where they catch the rising hot air and start their journey again; up, across the ceiling, fight for 90° space, sink down a little, etc.
And they’re still carrying their latent heat with them!
It’s reasonable to think that a steam molecule might make two or more loops like this before encountering some human skin in which to release its latent heat.
But Wait, There’s More – Following the Steam’s Trajectory
‘Sinking’ is complicated. The following images (Thanks to Corentin MacQueron and his modelling work in the latest publication on ResearchGate) show a computer simulation of the likely flight paths for a few 10 µm water droplets (in reality each one of these represents about a gazillion water droplets or maybe 17 gazillion steam molecules).
They follow the convective loop for part of their life but then, thanks to their own buoyancy and encountering the return air flow near the bottom of the convective loop, they often bounce up and fly around the upper reaches of our sauna for some period of time.
This first image is typical of saunas found in North America with two low benches. Note that the majority of the steam stays up in the convective loop above the top of the stones, a little gets down to sitting bench level, and none to our feet on the foot bench.
The sitting bench height in this sauna about matches the foot bench in my sauna from where the sauna slush chart above was produced. The correlation between this model’s prediction and the real data from our sauna is quite amazing.
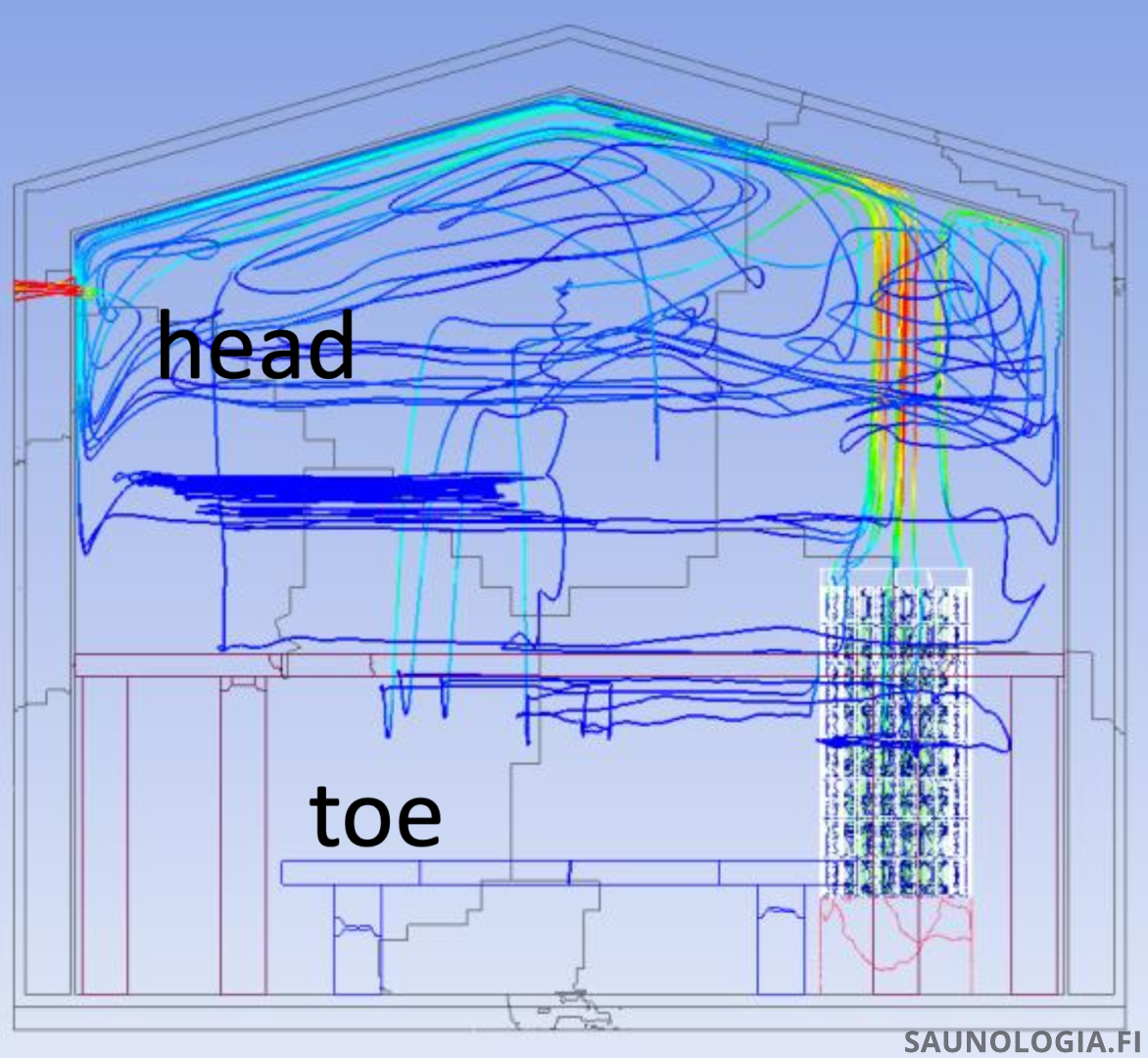
Next is a sauna with proper bench heights – Feet Above The Stones. Note that the steam is more evenly spread out generally thanks to the larger space above the stones but importantly is more even head to toes.
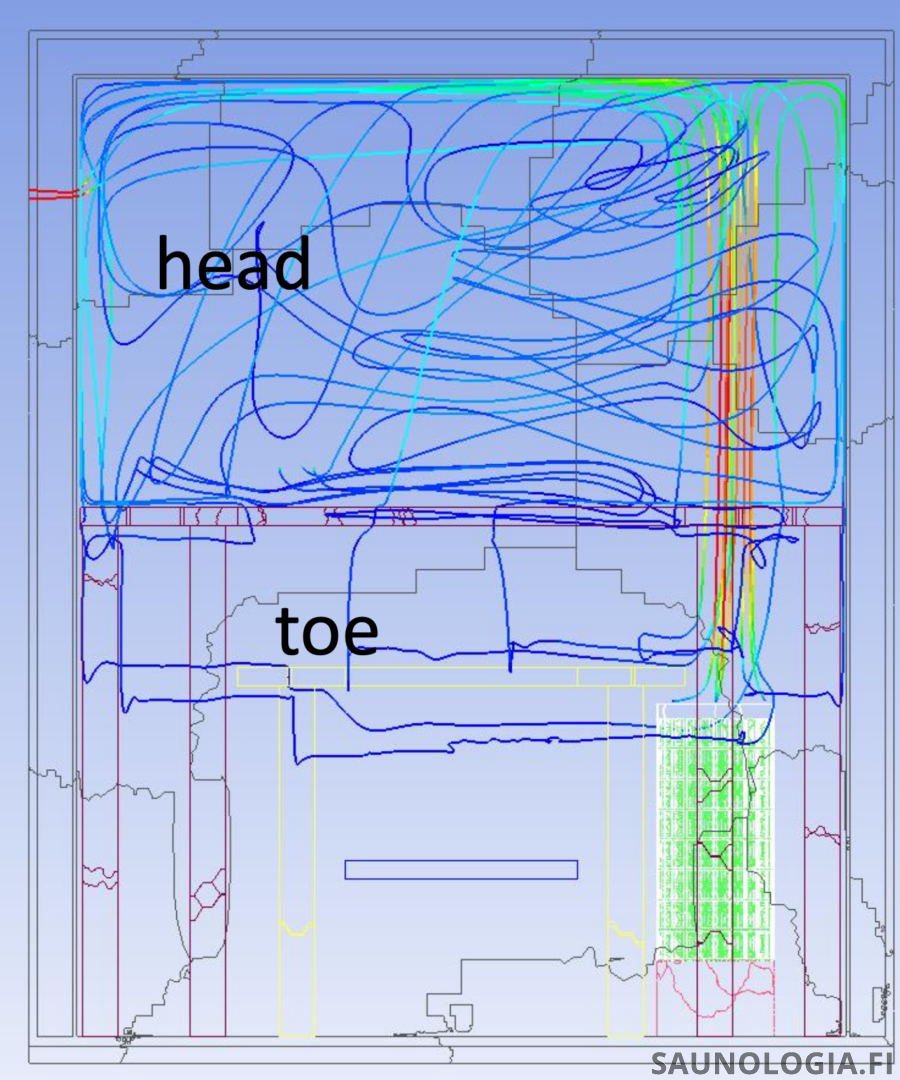
Below The Stones – Eventually the few molecules that remain will cool enough to sink through the bottom of the convective loop to the space below. As we saw in the sauna slush chart, this is after about 35-40 seconds in my sauna.
By this point there are simply not that many steam molecules left (the purple and green lines). The majority lived life up in the löyly pocket within the convective loop before condensing back to water. Of the few that make it down to the bottom of the convective loop only a few will sink below. And then, given the cooler air below the top of the stones, these molecules are more likely to encounter air below the dew point and condense into fog rather than release their latent heat in our skin.
A few also, rather than rising, will flow straight out from the heater towards us. We can see this where the purple line (sensor at about the bottom of our knee) rose up about the same time as steam made it down to our rump (yellow line).
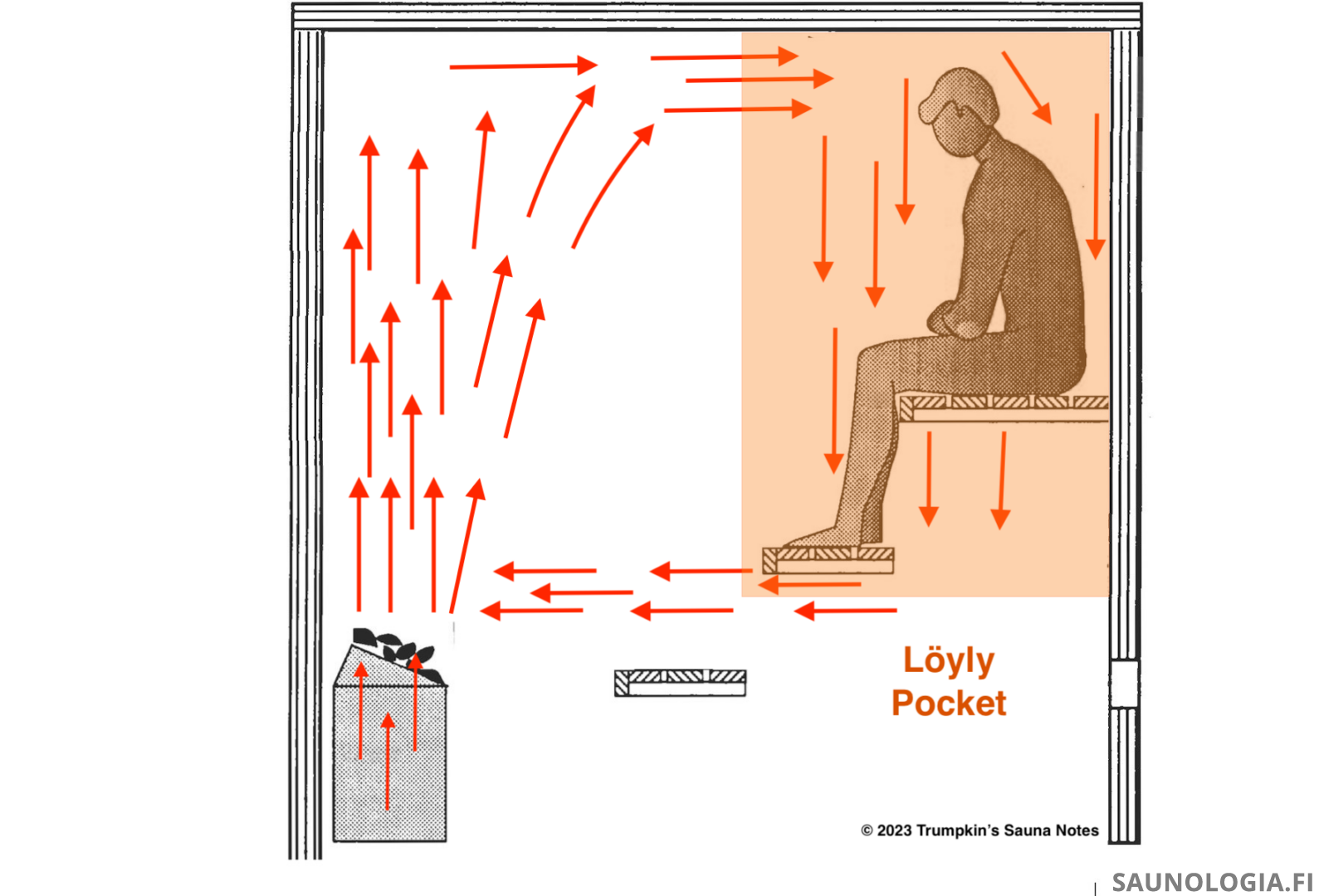
Open Mesh Tower Heaters – The return portion of the loop for these heaters appears to extend down further than it does for enclosed heaters which is why we’ll often see acceptable and sometimes even good temperatures lower down. The top of the return portion however appears to still be about 10-20cm above the top of the stones, which does little to compensate for their substantially higher build.
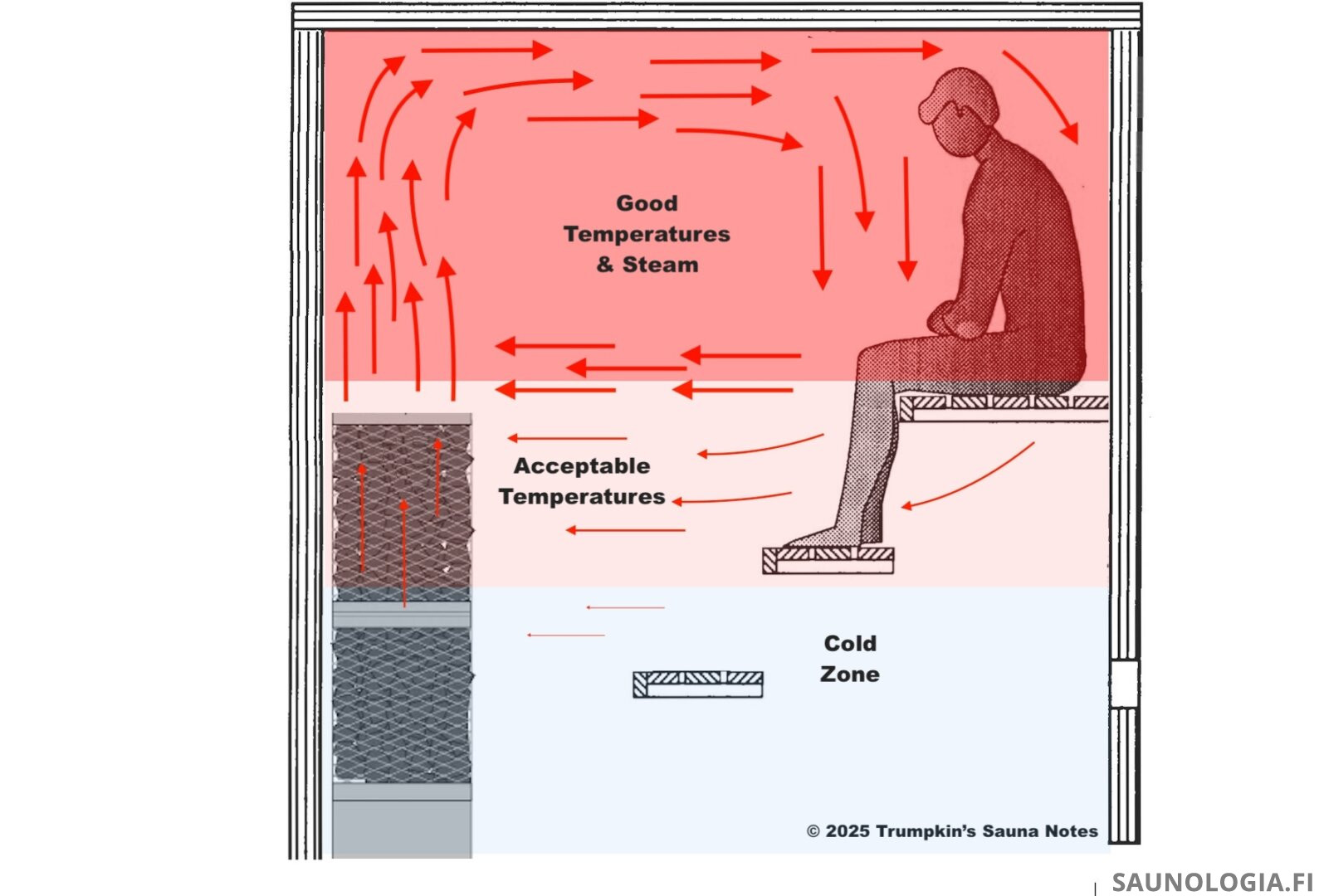
The steam molecules are likely catching a ride at the top of this return airflow. So we get good steam above this but little below it.
Why is air temperature stratification working differently than steam convective loop?
Why Doesn’t Downdraft Airflow Help With Steam As Much As It Does Air? – First is buoyancy and heat retention – steam wants, with considerable passion, to stay up high more so than air molecules do. So, it fights against downward air currents more than air.
This is very noticeable with aufguss. An aufguss master will fan the hot air and steam down but as soon as they stop, the steam quickly rises back up–it re-stratifies–while the air remains quite hot.
Critically though, there are many fewer steam molecules compared to air molecules, and they are smaller. With 24% relative humidity at 90°c, there is about 100 g/m³ of water vapor – steam molecules here make up only about 14% of the total molecules, while various air molecules, mostly N2, make up the other 86%. And the steam molecules are on average smaller.
Statistically then the steam molecules will have many fewer inter-molecular collisions, perhaps 1/20 as many, and so fewer downward collisions compared to larger and more numerous molecules like N2 that’s the primary component of air.
And that’s steam at fairly high density. As it becomes less dense in the air it will have even fewer collisions and be less affected by downward airflow (though also less buoyant).
So, a very powerful bit of airflow can increase the statistical likelihood of collisions and will often get steam to move down a bit, but only a limited amount and for a brief period.
In the end, if we want even steam head to toes, we have to be up in the löyly pocket above the stones.
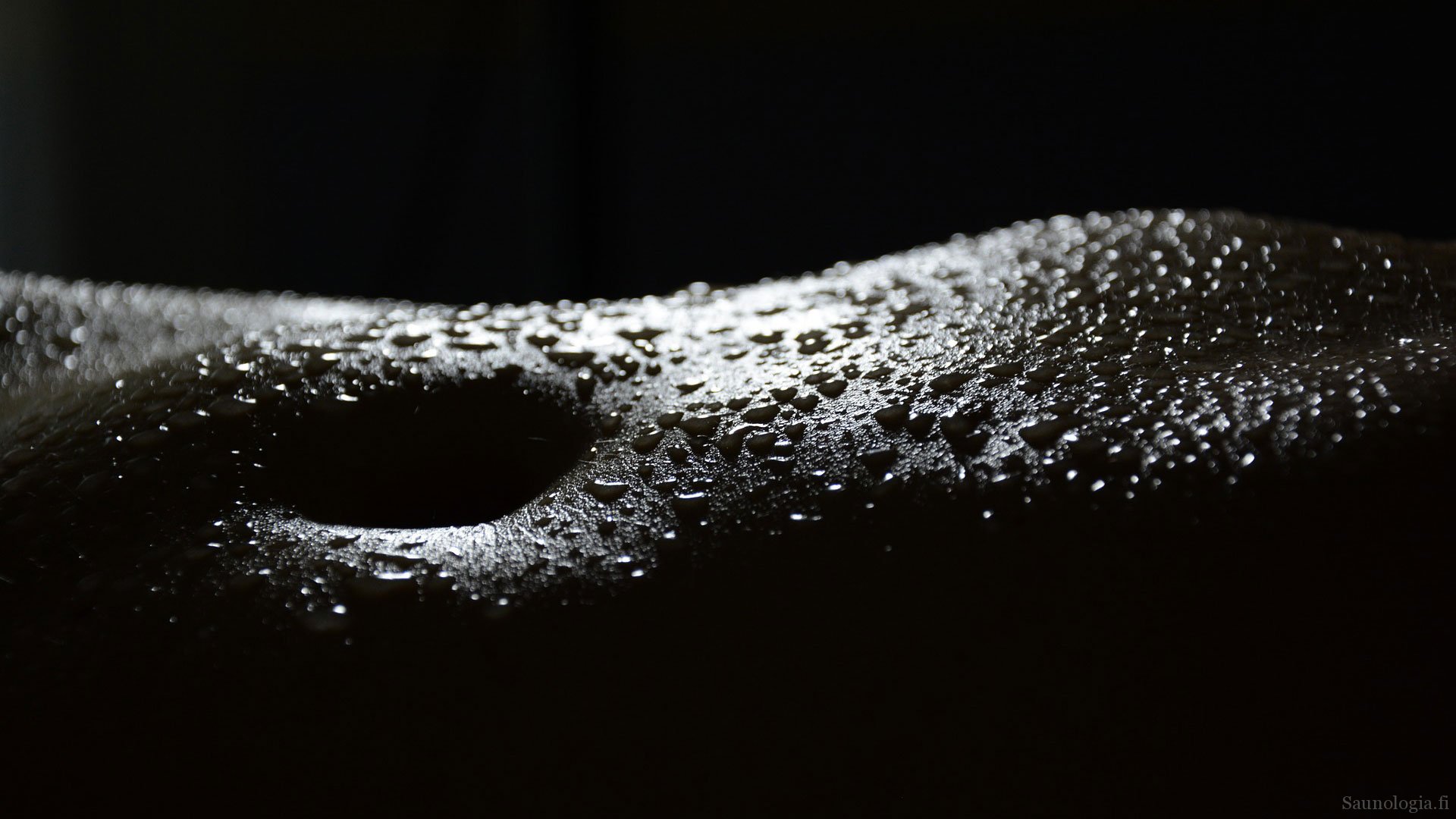
Factors working against steam free movement
Updraft Ventilation Hurts – While downdraft doesn’t help us very much with steam, it doesn’t hurt. Updraft ventilation however does.
First, since steam likes to remain higher, it is more amenable to going with the flow of updraft, which is not the direction we want it to go.
Second, since updraft ventilation brings cooler and drier air upwards, some of it likely below the dew point, then in addition to colder feet from these cooler air temperatures, we also have more of the steam condensing into fog, which has no latent heat potential, leaving less steam to condense and release its latent heat in our skin.
For those who just can’t get enough of this stuff… Consider this. When steam condenses in air to become fog droplets it releases its latent heat into the air which very minimally increases the air temperature. Some of these droplets then, when encountering our skin, can evaporate to become water vapor again, which steals heat from our skin to effect the phase change from liquid to gas – evaporative cooling of our skin.
Benches – Benches can cause the convective loop to shelve at the sitting bench rather than descend lower. This does seem to play a role with steam as well but we’re not sure how much of a role. This, and what’s needed for permeability, needs more exploration.
Conclusion
If we want a proper sauna experience with good even temps and steam and want to avoid cold feet then having our feet above the stones is still as critical today as Pälsi found it to be in 1960 and as it would have been in 1860.
The preceding is the result of numerous ongoing discussions amongst several people including Lassi, Ted, Corentin, Risto, Eero, Risto II and others about sauna dynamics. Here I am acting primarily as a documentarian of these discussions. Any errors are solely on me.
As an editor, I express my warm thanks for Walker in explicating some of the dynamics behind this decades old mystery, I am enlightened!
-Lassi
More Reading
From Saunologia:
Saunan lauteiden mitoitus ja löylyn laki [Finnish]
I should point out that experiments with hollowcore and other things are still ongoing.